Case study product development
Holistic virtual product development
The question is at which point a cycle begins. A cycle that has existed for many years in the context of virtual product development and has been customly accepted. But is this the right question? If we question this approach, new aspects consequently arise. For example, whether existing, previously proven mechanisms can or must be supplemented or extended in order to achieve set goals, perhaps even to exceed them, and if so, how?
The driving forces and potential for further development have become increasingly apparent in recent years, especially in the area of lightweight construction. This is not least due to the fact that the automotive industry is also under pressure to reduce CO2 emissions. Additionally, to manufacturing processes in fiber composite technology, injection molding processes and methods are also increasingly becoming the focus of a necessary change, a necessary progressive progress. On the one hand, the complexity of the products is increasing, and on the other hand, factors such as the necessary reduction of production steps, demanding design requirements and the highest expectations of the mechanical properties of the final product are the focal points of the manufacturing process.
One process that is increasingly coming into focus due to its potential is, amongst others, the foaming of plastics, meaning both chemical and physical foaming. The introduction of this innovative technology requires investments, on top of the obvious monetary ones, there are the ones for infrastructure, product development, staff training, upgrading of technical equipment and the resulting restructuring of work processes. In the beginning, for example, a mold originally designed for compact injection molding had to be adapted purely in terms of process technology. The adaptations were made resulting in a very different outcome and in some cases, were correspondingly diverse and often difficult to calculate. In favorable cases, weight reductions were indeed achieved. But there were also the negative cases, where the results did not meet expectations. The pros and cons of the "foaming" manufacturing process are therefore quite ambivalent. It is still a technology with potential, but also one in which a great deal of development and research effort still has to be invested.
But what is the actual situation regarding the development side of foamed components? Until today, tasks and steps are usually considered, analyzed and approached separately. On the one hand, the development of the product takes place directly from the designer to the mold designer. Additionally, a product development path leads to another department, which independently of the analysis and consideration of the process, performs calculations within the framework of mechanical simulation. Thus, the simulation of the manufacturing process usually takes place in product design and/or tool design. There, the fundamental challenge of making necessary adjustments to the process in the appropriate, effective work steps and sequences must be mastered, integrated as a matter of course. In the context of product design, for example, wall thickness ratios of base bodies and ribs have to be reconsidered. Adjustments are also necessary in mold design, for example with regard to connections.
However, for the product development it is also of interest of how foamed products behave under mechanical load and how they should be designed. Traditionally, these steps have so far been carried out in separate departments, a consequence of the different focus of the respective field of activity.
For some years now the focus has increasingly been on issues relating to the "foaming" manufacturing process, such as the processing of fiber-reinforced plastics. This process has enormous development potential, especially with regard to lightweight construction. The "usual" independent product development steps, which have already been described, can also be found here. But does this fully and effectively exploit the potential of the technology? Is a change of one's view necessary because it is more target-oriented? And if so, what are the technological and, above all, the time and financial implications of these adjustments?
To answer these questions, the first step is to take stock of the current status. Then one finds out that the tools needful for improvement often already exist. Likewise, the question is not how these tools are used. Rather, it is a question of how the results obtained are evaluated, how they are used, and how they are communicated and to whom.
One elementary aspect lies in the common approach to the mechanical design of products, whether made of fiber-reinforced plastics, by means of physical or chemical foaming or similar processes. This is because this method of consideration is usually based on the assumption of the same mechanical properties for the entire component. However, this is precisely the crucial point of the matter, as this approach significantly reduces and incompletely exploits the possibilities of these technologies, as they are only considered from one side.
What potential lies in the linking of these work steps - the consideration of the process and that of the mechanical properties - to a circuit concealed?!!! - In the unification of two sofar independent components in one cycle, in order to significantly supplement, exponentially improve the substance of virtual product development.
As trivial as it may sound today, a good basis for all steps lies in the consistency of the design data. The use of different CAD programs is still a source of possible resulting challenges. For example, the details of each CAD kernel (not CAD program) are not consistent and data import and export can result in an unwanted overlapping of surfaces or other errors. Since two different CAD programs are often used to generate product design and tool design this phenomenon usually occurs frequently. For the necessary rework or preparation of the data for the simulation, CAD programs as well as specialized software tools are available.
Process simulation provides the basis for overcoming possible challenges to be expected due to the manufacturing process, product design, mold design and/or the material of foamed components. The entire process from filling to cooling to simulation of deformation is calculated, analyzed, evaluated and optimized. Among the common simulation results of foaming are two in particular, which have a partly considerable influence on further development steps. One is the cell size distribution and the other is the cell density distribution. These two results not only allow conclusions to be drawn with regard to "cosmetic" issues. Rather, they reflect how pronounced the local edge layer is, whether more or less streaks are to be expected on the surface, and how high the expected deformation of the final component is. Furthermore, they also clarify process-relevant questions as to whether the use of variothermal annealing is advisable.
The decisive aspect for the successful coupling of two previously independent components - process simulation and structural mechanics - in a product development cycle now lies in the following step. For a long time, as already explained, these two methods were left out of the equation for the manufacture of safety-relevant products, since a continuous simulative process as well as mechanical simulation should not be possible. However, if some important aspects are taken into account, exactly this succeeds and, in addition, there is even a maximally usable positive effect. The description of the resulting cells can be transferred via porosity results from Moldex3D (simulation program used for process simulation) to the software tool Digimat for the purpose of material modeling. The resulting locally different material parameters are then passed on to the structural mechanics simulation. The relevance of this approach is illustrated in picture 1. Here, a stress-strain diagram is shown as a function of the microstructure.
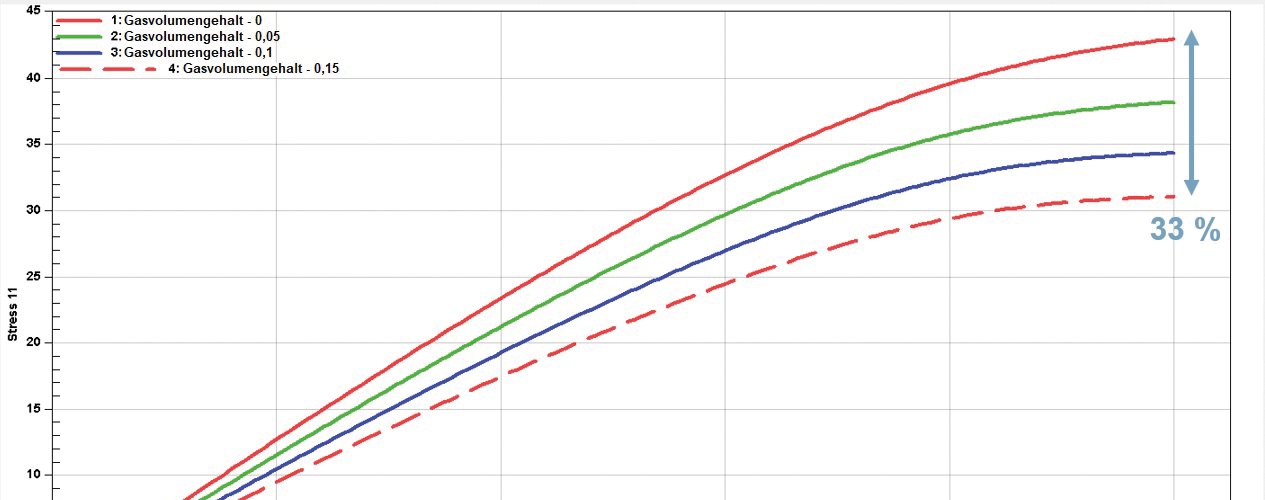
This ensures that the local different states of the material are taken into account accordingly and thus that targeted corrections of the component with regard to the mechanical properties can subsequently be realized. The subsequent verification of the geometric adjustments is again carried out by means of process simulation. Resulting, optimized process simulation results are repeatedly prepared for export to the mechanics. The cycle is closed. This process can be performed manually. However, there is also the possibility of a largely automatic optimization process. One advantage of optimization is that, in addition to the classic DoE approach, geometric adjustments are also made possible, up to and including automatic topology optimization.
Eventually, the question is how much is this effort worthwhile? Using a specific component, an orifice plate (see picture 2), the advantages will be presented in more detail.
Eventually, the question is how much is this effort worthwhile? Using a specific component, an orifice plate (see picture 2), the advantages will be presented in more detail.
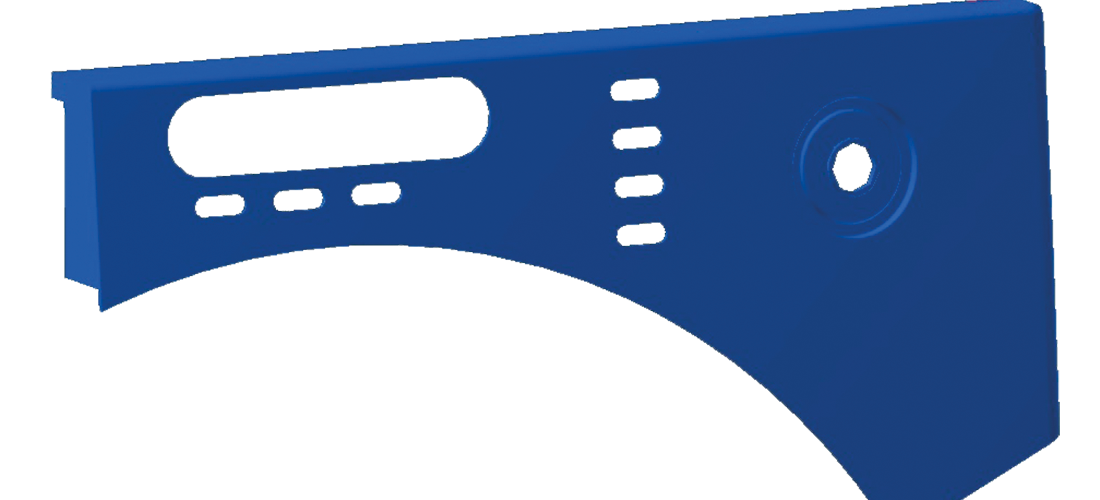
As part of the process simulation, the entire temperature control and distribution system was represented, calculated and analyzed in addition to the component, as can be seen here in picture 3.
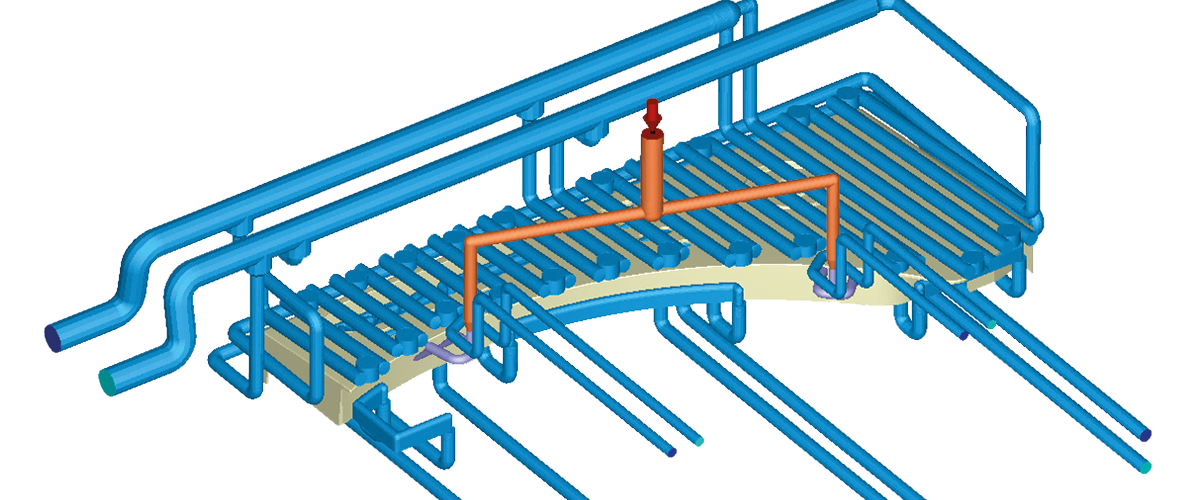
Picture 4 compares the simulated cell structure (left) with a CT image. A complete agreement between the calculated values and reality is evident. The relevant results of the porosity from the foaming process were then transferred to Digimat. With the material maps generated there, a three-point bending test was then calculated within FEA. In parallel, this test was also carried out with the real component.
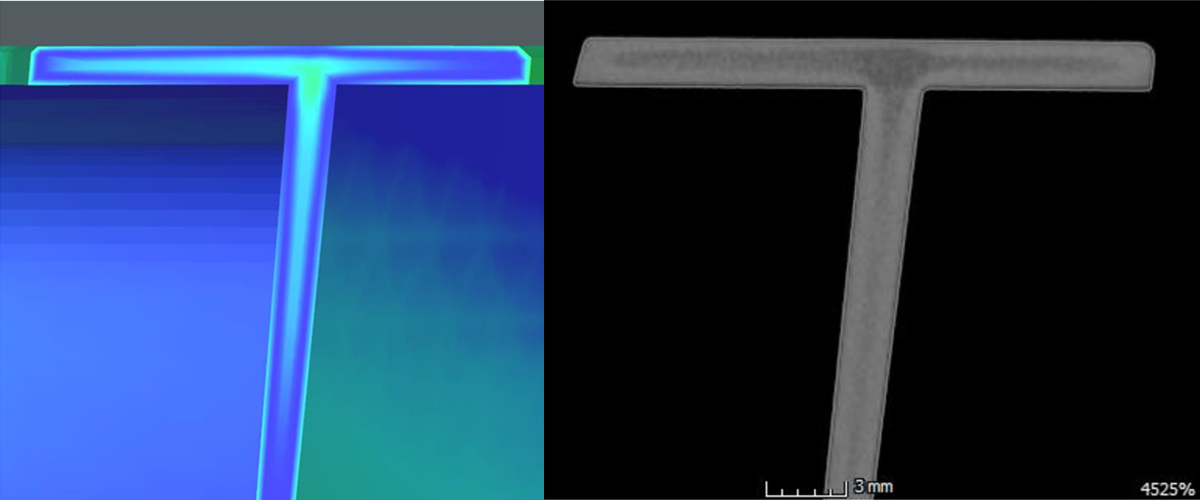
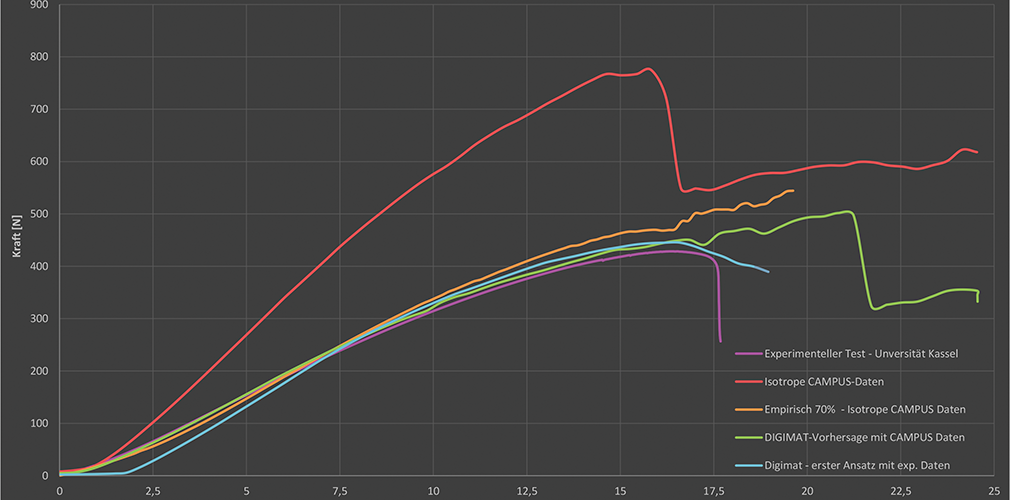
The resulting force/displacement diagram is shown in picture 5. It can be clearly seen here how much the isotropic approaches deviate from the real measurement (here in pink). This is not only true for the quantitative course, but also especially for the qualitative aspect of the failure. This diagram also shows the first results (in blue) of the continuous calculation, which represents a very good agreement with reality in both the qualitative and quantitative aspects.
Furthermore, optimization runs were also performed with the same product (the cover). The comparison began with the consideration of the compact injection molding process versus that of foaming. This already reduced the component weight by 10.1%. In a further step, the product design was optimized. The rib structure on the underside of the panel was optimally adapted to the required boundary conditions by means of 'sizing'. An adaptation step in accordance with the optimization based on mechanical properties. A further 5% reduction in weight was achieved. In addition, optimization of the manufacturing process was integrated into the product development process. Ultimately, thanks to the holistic approach and the advanced optimization approach, a weight reduction of 25% was achieved, with a simultaneous reduction in production-relevant deformations of a good 50% and a 10% reduction in the manufacturing cycle time.
Furthermore, optimization runs were also performed with the same product (the cover). The comparison began with the consideration of the compact injection molding process versus that of foaming. This already reduced the component weight by 10.1%. In a further step, the product design was optimized. The rib structure on the underside of the panel was optimally adapted to the required boundary conditions by means of 'sizing'. An adaptation step in accordance with the optimization based on mechanical properties. A further 5% reduction in weight was achieved. In addition, optimization of the manufacturing process was integrated into the product development process. Ultimately, thanks to the holistic approach and the advanced optimization approach, a weight reduction of 25% was achieved, with a simultaneous reduction in production-relevant deformations of a good 50% and a 10% reduction in the manufacturing cycle time.
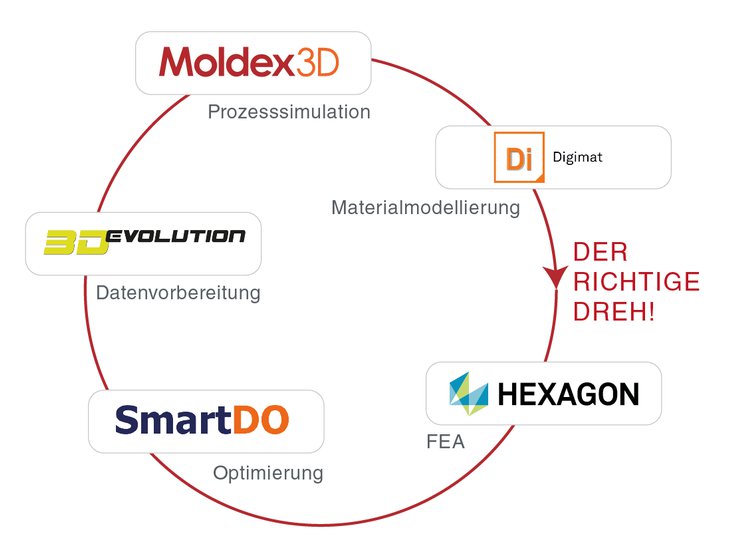
The holistic virtual development of a product (see picture 6) illustrates two essential aspects - firstly, with the help of the presented methodology, a holistic simulative consideration of foamed components is possible in a real and successful way. And secondly, it is quite obvious, with regard to the field of lightweight construction, that this approach conceals a potential which is just waiting to be exploited!
Acknowledgements:
We would like to thank:
_ the Institute of Materials Engineering of the University of Kassel for support in terms of material characterization and performance of the practical mechanical tests,
_ Ineos Styrolution Europe GmbH for the material provided,
_ the company F. & G. Hachtel GmbH & Co. KG for their support with the CT images and
_ Volume Graphics for the analysis of the CT images.
Acknowledgements:
We would like to thank:
_ the Institute of Materials Engineering of the University of Kassel for support in terms of material characterization and performance of the practical mechanical tests,
_ Ineos Styrolution Europe GmbH for the material provided,
_ the company F. & G. Hachtel GmbH & Co. KG for their support with the CT images and
_ Volume Graphics for the analysis of the CT images.